“One day, semiconductor demand and supply will match up – for all of 30 minutes.”
– attributed to Andy Grove, former CEO of Intel (1987 – 1998)
It’s difficult to think of an aspect of our daily lives that doesn’t involve a semiconductor somewhere. From the device you’re using right now to read this to the refrigerator you’re about to raid for a snack, from the smart television you use to binge-watch your favorite series to the traffic lights helping you get home safely every evening, there is nothing in your life that isn’t made possible by some finely etched bits of silicon.
In recent years, the world has discovered just how fragile the semiconductor supply chain can be, and what it means for every aspect of the modern global economy when the supply of semiconductors is disrupted. Disruptions to the flow of semiconductors caused even bigger bottlenecks in car manufacturing. A Deloitte study estimated that the 2020-2021 chip shortage caused $210 billion in lost sales for the automobile industry alone – to say nothing about other industry sectors.
More recently, the U.S. government has realized the full strategic, economic, and even military implications of depending on a globalized semiconductor industry that features single-company chokepoints at various points of the production process and/or supply chain. This is especially true when those chokepoints are located outside of the country and near rivals such as China or North Korea.
These two developments – supply-chain interruptions and a growing regulatory impetus to reshape the industry for strategic geopolitical advantage – are likely to reshape the industry in the coming decade. However, before we discuss those implications, let’s take a look at where we are now, and how we got there.
The Basics
In scientific terms, a “semiconductor” is a material that can conduct electricity under specific, controllable conditions but otherwise behaves like an insulator. This makes it enormously useful for the binary on-off functions of modern computing. In this sense, the first semiconductors were transistors: small rectangles of semiconductor material attached to an electronic circuit through several pairs of terminals, used to switch or amplify electronic signals.
Transistors made by Raytheon Corp., circa 1951.
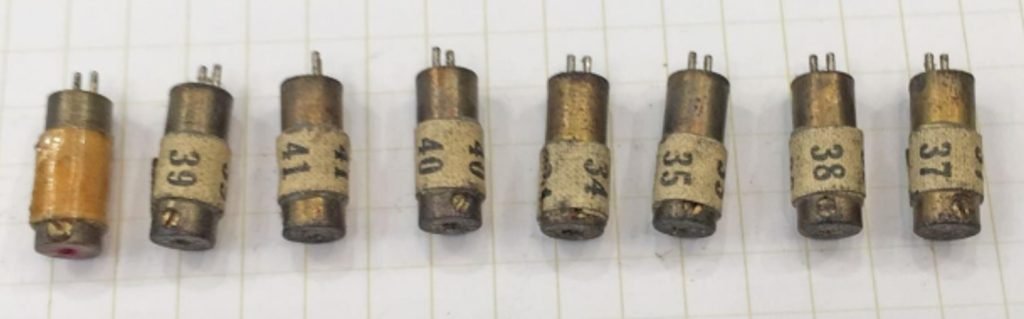
This led to the development of the integrated circuit, which packed multiple electronic circuits onto a single flat chip of semiconductor material.
Released in 1962, the Texas Instruments SN514 was the first commercially available integrated circuit
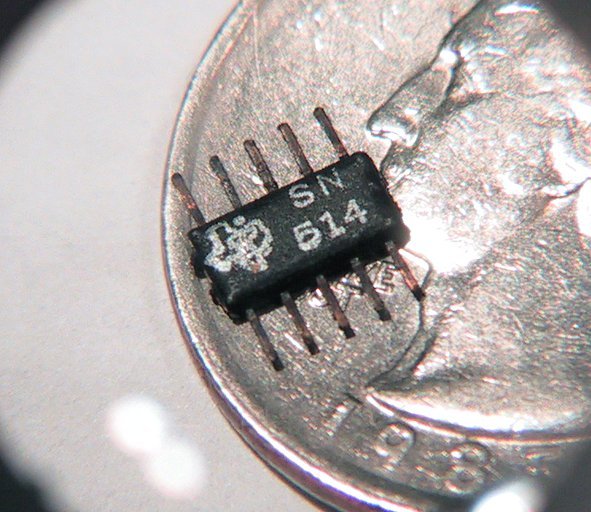
Metal-oxide-semiconductor (MOS) technology soon made it possible to put an ever-increasing number of circuits onto a single chip. And when we say “ever-increasing,” we mean it: Moore’s Law, posited by Intel co-founder Gordon Moore, co-founder of Intel, once predicted that technological advances would enable the maximum number of transistors on a chip to double every year. In 1975, he revised this time period to every two years. This prediction has held more or less true ever since. Today, a single chip can have millions or even billions of transistors, frequently 10 nanometers wide or less. (For reference, the coronavirus responsible for the COVID-19 pandemic is roughly 125 nm.)
The modern semiconductor manufacturing process includes thousands of steps, but they can generally be broken down into six stages (not including design).
- Deposition. Wafers are sliced from ultrapure cylinders (“ingots”) of silicon and polished. Thin films of conducting, semiconducting, or isolating materials are deposited onto the wafer.
- Photoresist coating. Wafers are then covered with a “photoresist” – one of a group of materials that are sensitive to specific wavelengths of ultraviolet light.
- Lithography. Deep ultraviolet light or extreme ultraviolet light (depending on the wavelength) is projected through a reticle (a blueprint or pattern) and then through a series of lenses or mirrors that shrink and focus that pattern. When the light hits the photoresist, it degrades the coating in the intended pattern.
- Etching. By baking the wafers and then washing them with either a mix of gases or a chemical bath, the parts of the photoresist affected by the lithography process are removed, leaving a 3D pattern of open channels.
- Ion Implantation. The etched wafer is then bombarded with positive or negative ions, implanting them into the silicon to change the electrical conducting properties in specific places on the wafer. This is the part of the process that actually creates the transistors. At the end of this stage, the rest of the photoresist is removed.
- Packaging. At this stage, each wafer consists of somewhere between a few dozen to thousands of chips. In the packaging stage, a diamond saw is used to cut the wafer into individual chips (or “dice”), and each die is then placed into a casing. This casing includes the metal foils that will connect the die to other parts of the system, operational heat-dispersion structures, and protection for the die from physical threats such as light exposure, impact, moisture, foreign particles, etc.
- Testing. The completed chips are then tested to make sure they function within acceptable parameters before being shipped to device manufacturers for end-stage assembly.
The development and constant refinement of this process represent one of the most impressive engineering efforts in human history. But it has come at a literal cost: Moore’s lesser-known Second Law predicted that the cost of a state-of-the-art semiconductor fabrication plant would double every four years. In the 1990s, American semiconductor companies started running into this trend. The cost of new fabrication facilities started becoming increasingly prohibitive, and the various technologies involved became so specialized that funding and managing the research to continue pushing the envelope became ever more complicated and expensively Sisyphean.
State of the Industry
In the beginning, all semiconductor companies were “integrated device manufacturers” (IDMs). That is to say that they designed and handled manufacturing in-house. But the demands of advancing each of the technologies in the various stages of manufacturing, and of implementing those advances, were becoming increasingly challenging. For example, by the 1980s, lithography had become sufficiently advanced and delicate enough that a change in air pressure due to an incoming thunderstorm could distort light sufficiently to ruin a wafer. Jerry Sanders, co-founder of Advanced Micro Devices, once famously compared owning a fabrication facility to keeping a pet shark in your swimming pool. Like the shark, fabs were expensive to feed, took a lot of effort to maintain, and might end up killing you. Despite this, he also famously said: “Real men have fabs.”
That attitude gradually became the minority view as fab prices became a significant barrier to entry for new players. That is why pure-play foundries came into being. Morris Chang, formerly of Texas Instruments, had long asserted that the IDM model would become ever more inefficient. Dr. Chang advocated a pure-play foundry as a business model in which high volumes would result in higher yields per wafer (as measured by the number of dice that pass quality control) and where economies of scale would make investments in technological advances more affordable and feasible.
After he failed to get the top job at TI in the early 1980s, Chang resigned. The Taiwanese government jumped at the chance to tap his expertise, asking him to help build the island’s technology sector. Ultimately, Chang founded the Taiwan Semiconductor Manufacturing Company (TSMC), with help from the Taiwanese government – which cannily reasoned at the time that such a company could become so critical to the potentially essential semiconductor industry and global economy that other countries would be more motivated to raise a stronger objection to any attempts by mainland China to seize control of Taiwan.
Startup tech companies have enough trouble getting seed capital to hire engineers. Convincing investors to chip in enough money to also build a pricey fab is exponentially more difficult. The introduction of the “fabless” semiconductor business model thus directly increased expansion and innovation in the industry. Some of the most important semiconductor companies are fabless and could not have come into existence if they had needed to raise funds for their own foundries. NVIDIA, for instance, began as a few engineers working out of a Denny’s restaurant in San Jose (which at the time was quite a rough city.) Other fabless innovators include companies like Qualcomm and Broadcom. (AMD, whose founder bragged about having a fab, is now also a major fabless player.)
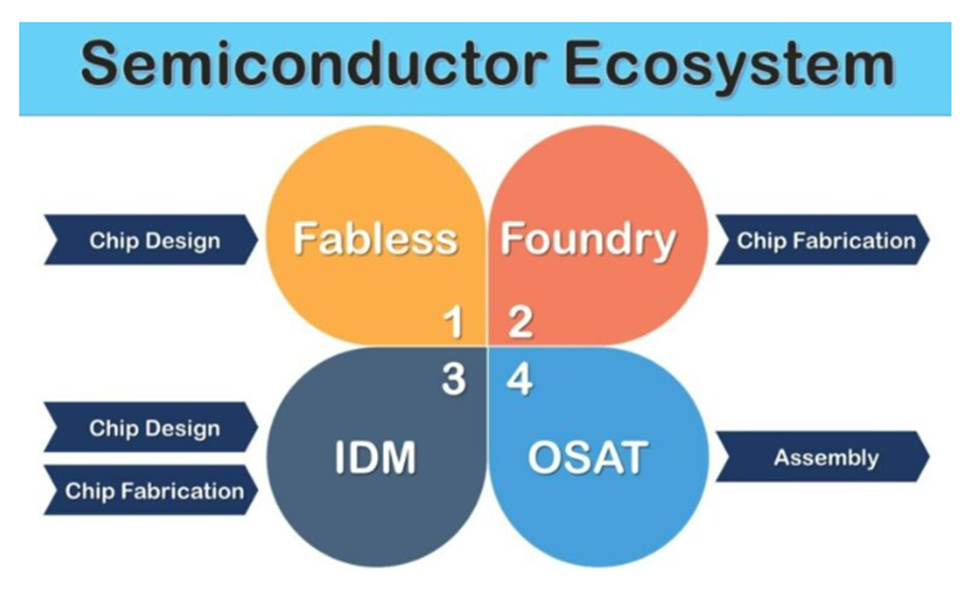
Today, TSMC is sometimes called the most strategically important company in the world, with a dominance in advanced semiconductor manufacturing that no other company can approach. It is worth noting that much if not all of the equipment used at TSMC comes from outside vendors. TSMC’s superiority stems not from the machines it uses, but from its advanced facilities and process expertise, particularly when it comes to proprietary algorithms used to coax maximum yield from cutting-edge materials and machines – honed from experience acquired while working with multiple clients on numerous projects. It doesn’t hurt that labor costs in Taiwan are much lower, of course.
That is not to say that TSMC is the only foundry business. South Korea’s Samsung and U.S.-based Global Foundries also provide fabrication services that are very much in demand. The latter is notable for its status as a “trusted supplier” to the U.S. Department of Defense.
Nevertheless, along with TSMC, several other companies have come to form an oligopoly in the industry, with a few big names dominating market share for very specific, specialized equipment. For example, among TSMC’s suppliers is Netherlands-based ASML, a spinoff from Philips NV that has a lock (100% market share) on the most advanced lithography machines – those that use extreme ultraviolet (EUV) wavelengths. Other aspects of chip manufacturing also feature companies with commanding market share. Japan’s Tokyo Electron, for instance, has a 90% share of the market for machines used in the deposition process; Lam Research is a leader in etching equipment, while Applied Materials has a commanding presence in the manufacture of machines used in other parts of the process.
Current estimates put the cost of a new fab at as much as $20 billion, and they take up to three years to complete. Still, despite the expense of building and running a fabrication facility, IDMs remain hugely important in the industry. The most well-known of these is Intel, still dominant in supplying the logic processors used in PCs and servers. Other major IDMs include Samsung, Micron, Texas Instruments, Analog Devices, and ON Semiconductor (aka onsemi).
As you will recall, we said that TSMC has by far the most advanced fabrication capabilities in the world. That includes the most well-known IDM household name, Intel, as well as the electronics conglomerate, Samsung. The reason we reiterate this is to note that not all vital semiconductors require the most advanced fabrication. That is mostly reserved for logic chips – processors, graphics processing units (GPUs), and Systems on a Chip (SoCs). These semiconductors serve as the brains of electronic devices like computers, smartphones, servers, and research supercomputers.
But Samsung and Micron make memory chips. Analog Devices, Texas Instruments, and onsemi make analog chips (which handle tasks like radio communications, power management/storage, and the processing of real-world audio and optical information). All of these are also vital, and they do not require TSMC’s bleeding-edge fabs to manufacture. (To be clear, TSMC is also more than able and willing to make those less-advanced semis for its clients, and it does a brisk business doing so.)
Chain, chain, chain …
The semiconductor industry has long had a problem with boom and bust cycles, frequently finding itself with overcapacity and oversupply during lean times and the reverse during times of high demand. The historically cyclical nature of the business had a lot to do with this. Semiconductors were once primarily or even exclusively used in computers and servers (and later, mobile phones), and they were affected by demand for those end-products, which tended to align with the state of the economy. Consumers tended to put off purchases of computers and phones when their personal finances were uncertain.
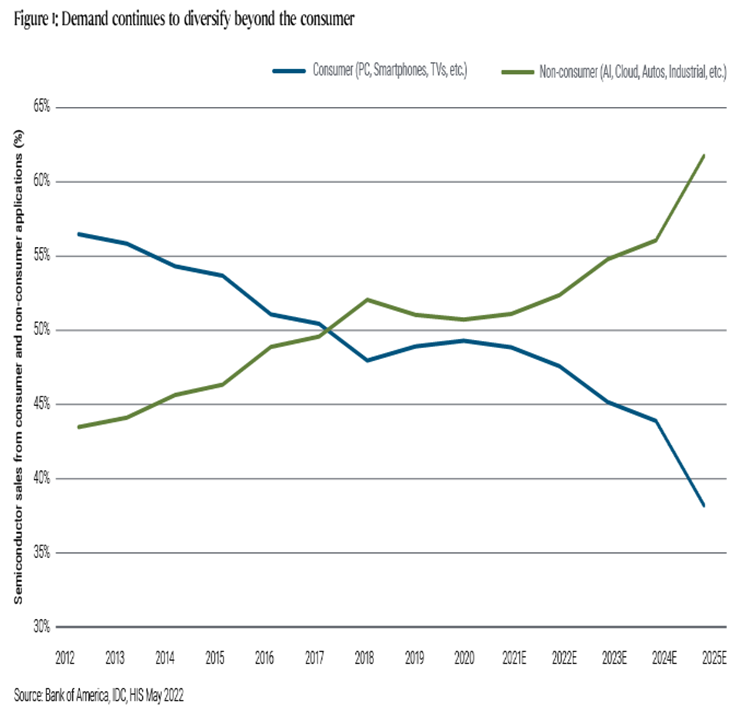
Some observers believe semiconductors are becoming less cyclical and will soon edge into non-cyclicality. Computers and phones are now regarded less as special purchases and more as necessities that should be immediately replaced whenever necessary, regardless of all but the direst financial circumstances. Furthermore, semiconductors have found their way into an ever-broadening array of products, including both cyclical and non-cyclical categories. Much has been written about the integration of semiconductors into modern automobiles (as many as 1,000 chips in each vehicle), but semiconductors are now also operationally critical to companies in the energy, defense, agricultural, healthcare (medical devices, pharmaceutical development), and utilities sectors. In time, the trend could help semi companies to better anticipate and manage supply and demand.
Still, some of the challenges of managing this remain tied to the nature of the manufacturing process. After a chip design is finalized, it takes as long as three months to set up all the steps in the manufacturing, assembly, and testing process, and not until the setup is complete can manufacturing even begin.
Furthermore, according to the Semiconductor Industry Association, the globalized manufacturing process includes more than 50 points where one region holds more than 65% of the market share. As the world found out during the pandemic and subsequent recovery, the potential bullwhip effect is massive when so many moving parts are involved.
In addition, that’s at least 50 places where any number of natural (earthquakes, a perennial threat in the Pacific Rim, have disrupted TSMC operations on multiple occasions) or manmade disruptions can hold up the process.
Regulatory changes
China
The implications of China’s pending rise and industrialization were not on the minds of U.S. government officials in the early 1990s when the tech sector was evolving into what it is today. The United States had just won the Cold War and was unquestionably the world’s only superpower. The industry, still almost entirely located in Silicon Valley, had just survived a threat from competitors in Japan – with a little help from friends in Washington.
The pro-globalization view was that as long as it wasn’t a militarily essential technology (and semiconductors at the time were not widely viewed as such), dispersion of technology would not be detrimental to U.S. interests – and in helping to promote trade and encourage democracy, might even be good for the United States.
But China’s rapid, broad modernization has led to implications that were likely not considered by officials at the time.
The world’s response
There are valid reasons to be concerned about three aspects related to China’s new and growing place in the world: the country’s quest to become a leader in supercomputing and artificial intelligence, the likelihood it might displace current players in vital parts of the semi manufacturing process and achieve dominance, and the geopolitical implications associated with those two possibilities and with China’s regional aspirations.
The United States on October 7, 2022 put a ban in place that restricts the sales to China of any advanced chips, tools, or components that could be used to make chips with node sizes of 14 nm or less. That includes not just chips, but also design software, chip manufacturing equipment, and components thereof. As the think tank CSIS put it, this policy was akin to “strangling with an intent to kill” China’s tech sector.
The ban is new enough that companies are trying different ways to respond. NVDIA, whose best GPUs are easily repurposed for supercomputing and AI research, on November 7, 2022 announced that it would release a special Chinese-market processor, the A800, that has been designed to be lower-performing to comply with the export ban.
Both the United States and the European Union have also put in place initiatives to try to incentivize fabrication capabilities, though these have as much to do with trying to achieve self-sufficiency for domestic industries and manufacturing. The U.S. CHIPs act, signed on August 9, 2022, provides $52.7B in grants, loans, and loan guarantees over five years to incentivize domestic semiconductor manufacturing. The incentives include a 25% tax credit for investments in fabrication, but also one provision: beneficiaries would be prohibited from expanding in China for 10 years (excluding legacy projects.) Companies taking advantage of this incentive include Micron, Qualcomm, and GlobalFoundries.
Other challenges
Commodities: The semiconductor industry is sensitive to price fluctuations in several key commodities. It begins with energy prices: semiconductor manufacturing is extremely power-intensive. TSMC, for instance, is the single largest consumer of power in Taiwan, accounting for 7% of the island’s demand. The manufacturing process also requires the use of certain gases to create a chemically inert environment. Several of these, like helium and neon (half of the world’s supply) are (or were) purified by two Ukrainian companies. Germanium is another key ingredient in the process, and 90% of the global reserves of this metal are located in Russia and China. Any geopolitical or environmental events that affect the supplies or prices of materials like this will affect costs for all members of this industry.
Labor: Nativists have long questioned why tech manufacturing had gone overseas, blaming companies for sacrificing domestic jobs just to save on labor costs. And while lower labor costs are certainly an attractive consideration, the real problem had to do with a dearth of skilled labor. The United States simply does not produce enough electrical engineers and industrial engineers needed to move the manufacture of any advanced technological product – whether a logic processor or an iPhone – domestically. Companies have tried: TSMC in August 2022 had to delay the opening of a fab in Arizona by six months in large part due to its inability to hire enough workers. (The fab is scheduled to begin manufacturing in 2024.)
Environmental: In addition to consuming a lot of power, the industry also consumes a lot of ultra-purified water, used to rinse chemicals away during and after the manufacturing process. To illustrate TSMC accounts for 10% of Taiwan’s water usage – 70 billion liters, or 18.5 billion gallons, every year. This raises both financial and ESG-related concerns that semiconductor companies have acknowledged and will be seeking to ameliorate in the coming years.
Technology/physical limits: Naysayers have been saying for years that we were approaching the limits of Moore’s law. Finally, they might be about to be vindicated. Today’s most advanced chips have transistor gates that are just 2 nm wide. That’s just 10 times the width of a silicon atom (0.2 nm). Smaller transistor gates will start butting up against the fundamental laws of physics: smaller gates might be insufficient to do their job of containing and controlling the flow of electricity through a chip. And yet, that fundamental physical principle might be circumvented if, rather than trying to cram more transistors in a 2D fashion, chip designers start thinking in 3D, stacking layers upon layers of dice on top of each other. The challenge to this approach stems from the complexity of stacking chips on a mass scale and finding a way to disperse the heat that stacks of dice would generate during operation. As of this writing, researchers have proof of concept supporting the validity of the 3D stacking approach, but its viability for mass production remains to be determined.
Our Favorite Semiconductor Stocks
Although the semiconductor industry faces multiple significant challenges as detailed above, the looming ubiquity of the semiconductor means excellent prospects for established companies in this sector. Some of the stocks we like include:
Qualcomm (QCOM).
Qualcomm played a key role in the early days of mobile phones, helping to establish the protocols and standards that mobile networks would deploy. Its influence in mobile networks and devices, including smartphones, has continued to expand. The company has warned of pressures in the near term owing to anticipated lower demand for handsets (particularly in China), but its involvement in Internet of Things devices and automobiles are positives that should not be overlooked. Qualcomm’s strong presence in the field of Artificial Intelligence research also bode well for its future.
ASML (ASML).
Any company – whether it is Samsung, TSMC, Intel, or someone else – that wants to make the most advanced semiconductors needs to buy EUV lithography machines from ASML: there is no alternative on the market. Because of this monopoly and because any company’s purchase of an ASML lithography machine is always part of its long-term plan, the Dutch company is somewhat insulated from the cyclicality of what has historically been a cyclical industry.
NVDIA (NVDA)
As the quality of computer graphics improved, the processing power needed to render them on a screen rose to the point where it started to make sense for devices to incorporate a dedicated graphics processing unit. GPUs are well suited for this task because they are designed to process large volumes of very basic calculations simultaneously – for instance, applying a shading algorithm to every pixel of an image. But it turns out that this sort of design is also very useful in AI research and will be critical to the development of virtual reality and Metaverse technologies.
ON Semiconductor, aka Onsemi (ON).
Arizona-based Onsemi is one of the leading manufacturers of analog chips. The IDM’s product lines focus on power management (helping devices manage power and power storage) and optical sensors/signal processors. Onsemi solutions are widely used in the automotive (EV) and industrial sectors. This focus will likely be advantageous for long-term growth and profits. Another area of focus for the company is sensor chips. The proprietary tool developed by FS Insight Head of Quantitative Strategy Adam Gould currently rates Onsemi as a strong outperform.
TSMC was previously discussed in detail in the October 15, 2021 edition of Signal From Noise. It remains a strong investment option in the semiconductor industry. Also previously in SFN (September 24, 2021) was Texas Instruments (TXN), which remains a company with a strong long-term outlook.